- Home
- Enhanced Cryopreservation of T Cells Using Optibumin®, Recombinant Human Serum Albumin (rHSA)
Enhanced Cryopreservation of T Cells Using Optibumin®, Recombinant Human Serum Albumin (rHSA)
Published on 21 January 2025
Application Note
Andrew Hamann PhD, Product Applications Scientist, InVitria, Inc.
Mark Stathos PhD, Product Applications Scientist, InVitria, Inc.
Key Points
Optibumin® Improves T Cell Recovery and Proliferation Post-Thaw
- Optibumin significantly enhances T cell viability and proliferation post-thaw compared to human blood-derived clinical HSA.
Reduction of DMSO Concentrations in CryoStor® Formulations
- The inclusion of Optibumin solution physically displaces an equal volume of CryoStor to enable a reduction in final DMSO concentrations, improving the safety profile of final formulations.
Preservation of Key T Cell Memory Phenotypes
- Cryopreserved cells retained high proportions of stem cell memory (Tscm) and central memory (Tcm) phenotypes post-thaw, crucial for CAR-T therapy efficacy.
Mitigation of CD8+ T Cell Loss During Cryopreservation
- The inclusion of Optibumin in CryoStor CS10 formulations preserved CD8+ cytotoxic T cell populations, helping to maintain a balanced CD4/CD8 ratio.
Introduction
Cryopreservation is a pivotal step in the manufacturing of T cell-based therapies, directly influencing cell viability, functionality, and clinical outcomes post-thaw. Optimizing cryopreservation protocols is critical to maintaining T cell potency and ensuring consistent therapeutic performance. While human serum albumin (HSA) is commonly used to enhance cell health and functionality post-thaw (Burnham, 2021) and is included in several on-market cell therapies (Walle, 2021), blood-derived HSA presents potential risks, such as pathogen transmission (MacLennan & Barbara, 2006) and adverse reactions (Lu, 2024). Regulatory agencies increasingly recommend animal-origin-free materials to address these safety concerns and support reproducibility in therapeutic production (U.S. FDA, 2024).
InVitria’s Optibumin, a recombinant human serum albumin (rHSA) offers an animal free, scalable, sustainable, and cost-effective solution. Optibumin eliminates risks associated with blood-derived products, ensures batch-to-batch consistency, and supports regulatory compliance. Optibumin is manufactured under cGMP conditions in an ISO9001:2015-certified facility located in the USA, making it ideal for clinical-grade applications in cell therapy manufacturing.
Optibumin is specifically engineered for seamless integration into existing cell manufacturing workflows. Offered as a 25% solution in convenient bags, it is ideal for closed-system processes. Optibumin can be easily mixed with pre-formulated cryopreservation media, such as CryoStor CS10 or CS5 ensuring ease of adoption while delivering exceptional safety, performance, and compatibility with the demands of closed-system manufacturing.
This application note demonstrates the benefits of Optibumin for T cell cryopreservation. In this study, albumin solutions were added to chemically defined and protein-free CryoStor CS10 and CS5 cryopreservation media. By partially replacing DMSO-containing CryoStor media in final formulations, this approach reduces DMSO concentrations while enhancing post-thaw performance. Key benefits include improved T cell viability and proliferation while retaining key memory phenotypes such as stem cell memory (Tscm) and central memory (Tcm). Optibumin was directly compared to a clinically used, human blood-derived HSA, with Optibumin demonstrating superior post-thaw recovery and proliferation. This study not only confirms Optibumin’s effectiveness, but also its seamless integration into cryopreservation workflows, making it a reliable and innovative solution for next-generation cell therapy manufacturing.
Results and Discussion
Optibumin Improves Post-Thaw Viable T Cell Proliferation with Reduced DMSO
In Figure 1, the post-thaw proliferation of human T cells from two healthy adult donors was assessed after cryopreservation in CryoStor CS10 (10% DMSO) or CS5 (5% DMSO) formulations containing either 0%, 5%, or 10% albumin (Optibumin rHSA or a clinically used HSA). Cells were plated in growth medium immediately after thawing, and the number of viable cells was normalized to the first timepoint and monitored over 72 hours. Across all conditions, T cells display a decline in viability during the first 48 hours post-thaw, reflecting the cellular stress induced by cryopreservation and thawing. This decline was most pronounced in conditions without albumin, where viable cell counts dropped to their lowest levels (i.e., about 50% of initial) before recovery began.
The inclusion of albumin significantly improved T cell recovery and proliferation post-thaw, with Optibumin formulations consistently outperforming clinical HSA. In CryoStor CS10 (Figure 1A and 1C), 10% Optibumin enabled approximately two-fold expansion of viable cells after 72 hours, followed closely by 5% Optibumin. Statistical analysis revealed that for both donors, Optibumin at both 5% and 10% concentrations resulted in significantly greater expansion compared to blood-derived HSA (p-value < 0.001). By comparison, blood-derived HSA at 10% offered moderate benefits, while 5% blood-derived HSA showed only limited improvement over the no-albumin control. Notably, Optibumin consistently outperformed across both donors, underscoring its reliability and effectiveness in enhancing cell recovery and proliferation.
In CryoStor CS5 (Figure 1B and 1D), the benefits of Optibumin® remained evident, though slightly less pronounced compared to CS10. For Donor 1, 10% Optibumin achieved a 1.6-fold expansion, while Donor 2 showed a 1.25-fold expansion. Statistical analysis confirmed 10% Optibumin resulted in significantly greater cell expansion compared to blood-derived HSA (p-value < 0.05) for both donors. In contrast, blood-derived HSA provided little benefit in CS5, with proliferation curves closely resembling those of the no-albumin controls. These findings highlight the superior performance of Optibumin in formulations with reduced DMSO concentrations, where blood-derived HSA was notably less effective.
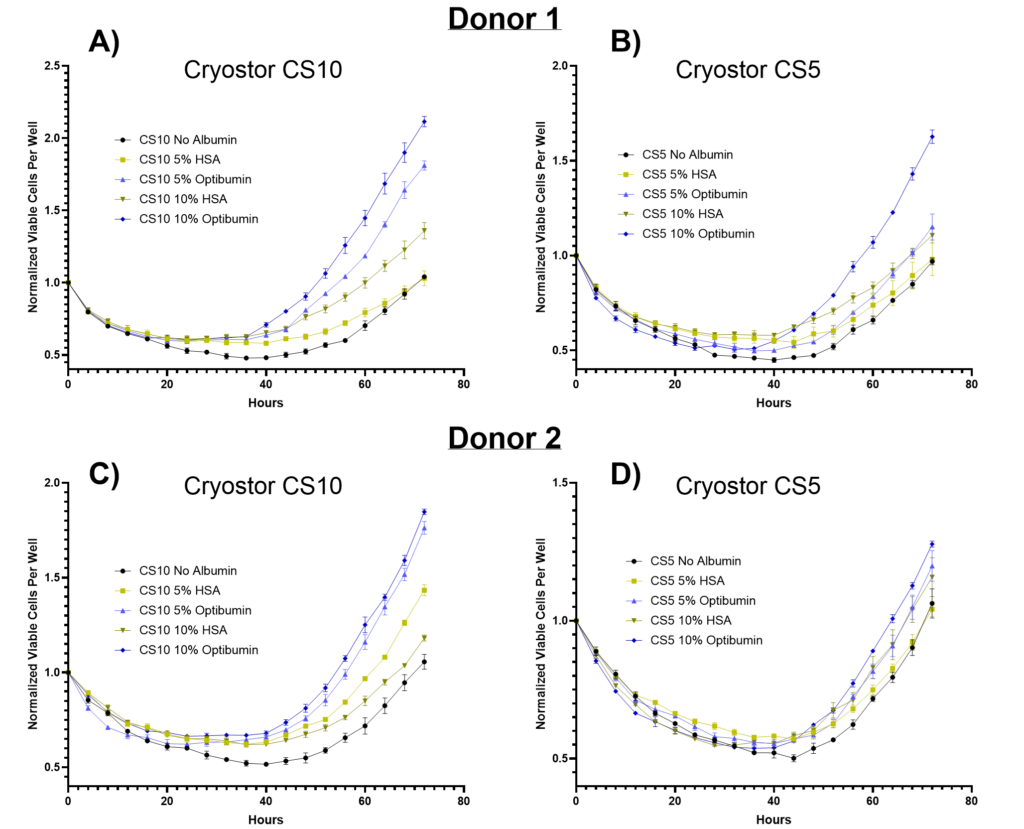
In addition to enhancing cell recovery and proliferation, the inclusion of albumin (provided as a 25% solution) actively reduces the final DMSO concentrations in the cryopreservation formulations used in this study. This reduction in DMSO is a result of the albumin solution displacing an equivalent volume of CryoStor. As shown in Table 1, incorporating albumin at 5% and 10% reduced DMSO levels from 10% to 8% and 6% in CryoStor CS10, and from 5% to 4% and 3% in CryoStor CS5, respectively. These reductions are important for clinical applications, as high DMSO concentrations are associated with adverse reactions in patients upon infusion (Madsen, 2018). By providing both superior post-thaw outcomes and a lower DMSO burden, Optibumin demonstrates clear advantages for optimizing cryopreservation workflows in T cell therapy manufacturing.
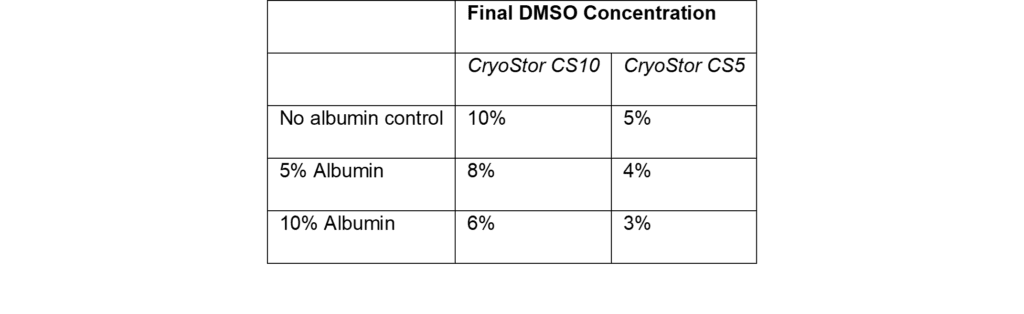
Preservation of Early Memory T Cell Phenotypes Post-Thaw with Cryopreservation Formulations
In Figure 2, the proportions of T cell memory phenotypes were assessed 24- and 72-hours post-thaw for T cells cryopreserved in CryoStor CS10 or CS5 with varying albumin formulations. Early memory phenotypes (stem cell memory (Tscm) and central memory (Tcm)) are critical for the efficacy of CAR-T therapies due to their superior proliferation, persistence, and anti-tumor activity (Fazeli, 2023; Meyran, 2023). In contrast, more differentiated phenotypes (effector memory (Tem) and terminal effector (Temra)) exhibit limited persistence and proliferative potential, making them undesirable for therapeutic applications (Arcangeli, 2022; Lin, 2023).
At 24 hours post-thaw (Figures 2A and 2C), cryopreserved cells displayed higher proportions of early memory phenotypes (Tscm and Tcm) compared to pre-cryo samples. This trend was observed across all cryopreservation conditions, regardless of albumin inclusion, which may be due to increased susceptibility of differentiated phenotypes (Tem and Temra) to cryo/thaw-induced stress. Notably, over 80% of cells from all cryopreserved conditions retained early memory phenotypes (Tscm and Tcm) at this timepoint. In contrast, the unfrozen control exhibited slightly higher proportions of differentiated phenotypes (Tem and Temra), likely due to its extended culture time relative to the cryopreserved samples.
By 72 hours post-thaw (Figures 2B and 2D), the proportion of early memory phenotypes (Tscm and Tcm) decreased across all conditions as cells continued to differentiate in culture. Approximately 50% of cells in the cryopreserved conditions had differentiated into later memory phenotypes (Tem and Temra), while the unfrozen control exhibited the highest differentiation, with 70% of cells in these later phenotypes. This differentiation trend reflects the natural progression of T cell phenotypes during in vitro culture after activation and emphasizes the need for careful control of manufacturing conditions to preserve early memory phenotypes.
Importantly, the inclusion of albumin (Optibumin or clinical HSA) had minimal impact on the proportions of T cell phenotypes at either 24- or 72-hours post-thaw. This suggests that while albumin provides significant benefits for post-thaw viability and proliferation (Figure 1), it does not significantly influence the differentiation trajectory of T cells in culture.
The persistence of early memory phenotypes (Tscm and Tcm) is critical for the long-term efficacy of CAR-T therapies, as these cells exhibit superior anti-tumor activity and persistence compared to more differentiated phenotypes. Maintaining these populations throughout the manufacturing process is especially important when working with patient-derived samples, where early memory T cell populations are often depleted (Das, 2019). These findings highlight the importance of optimizing cryopreservation and manufacturing conditions to preserve the desired T cell phenotypes for therapeutic success.
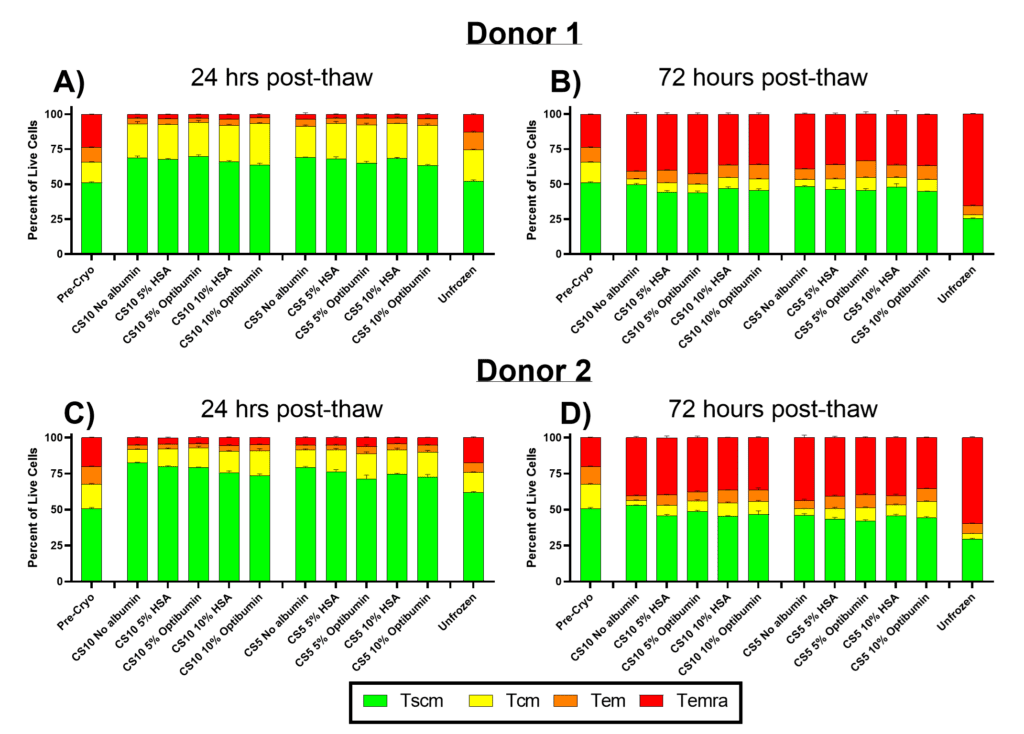
Maintaining CD4/CD8 T Cell Balance During Cryopreservation
In Figure 3, the proportions of CD4+ (helper) and CD8+ (cytotoxic) T cells were assessed at 24- and 72-hours post-thaw for T cells cryopreserved in CryoStor CS10 or CS5 with varying albumin formulations. The CD4/CD8 ratio is a critical parameter for CAR-T manufacturing, as it influences therapeutic efficacy. Elevated CD4/CD8 ratios are associated with poor outcomes (Galli, 2023), emphasizing the importance of maintaining a balanced ratio during manufacturing.
Pre-cryo, both donors displayed a CD4/CD8 ratio of approximately 3:1, with 25% of cells CD8+ and 75% CD4+. At 24 hours post-thaw (Figure 3A and 3C), all cryopreserved conditions showed a reduction in CD8+ cells, decreasing to nearly half of the original proportion. However, the addition of albumin, particularly in CS10 formulations, slightly mitigated CD8+ cell loss compared to the no-albumin controls (from 9% to 16% in Donor 1 and 13% to 20% in Donor 2). This effect may reflect albumin’s role in protecting cell viability during cryopreservation and recovery. In contrast, unfrozen controls maintained their pre-cryo CD4/CD8 ratios, potentially due to selective stress cryopreservation imposes on CD8+ cells.
At 72 hours post-thaw (Figure 3B and 3D), the CD4/CD8 ratios remained largely stable relative to 24 hours for donor 1, with little further reduction of CD8+ cell proportions. However, Donor 2 exhibited a slight decline in CD8+ proportions at this timepoint, potentially reflecting donor-specific variability in post-thaw recovery. Importantly, the inclusion of albumin continued to slightly preserve CD8+ proportions, particularly in CS10 formulations.
These results highlight the challenge of maintaining balanced CD4/CD8 ratios during cryopreservation, with CD8+ cells appearing more susceptible to cryo/thaw stress. The protective effect of albumin, particularly Optibumin, suggests it could help preserve cytotoxic T cell populations during cryopreservation, potentially improving the therapeutic quality of CAR-T cell products. Given the importance of balanced CD4/CD8 ratios for efficacy and patient outcomes, optimizing cryopreservation protocols to minimize CD8+ cell loss remains a critical focus for manufacturing next-generation cell therapies.
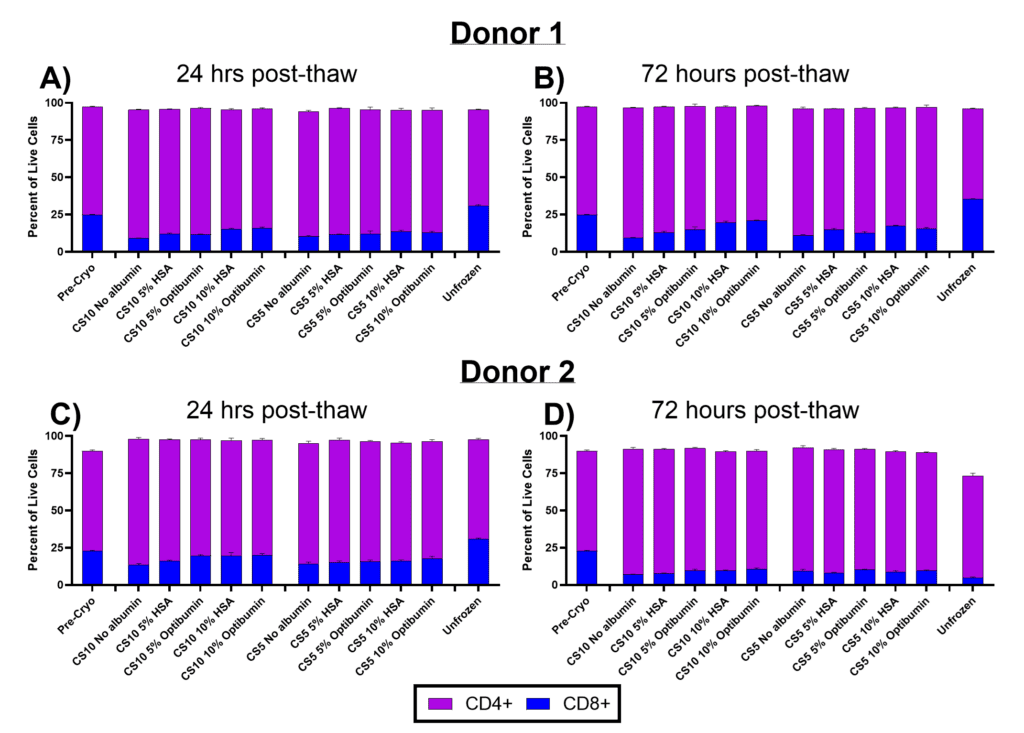
Conclusion
This study demonstrates the superior performance of Optibumin recombinant human serum albumin (rHSA) in T cell cryopreservation, offering significant benefits for cell therapy manufacturing. Optibumin consistently outperformed clinically used blood-derived HSA by enhancing T cell viability, proliferation, and post-thaw recovery, all while reducing DMSO concentrations to improve the safety profile of cryopreserved T cells.
Notably, Optibumin preserved critical T cell memory phenotypes (Tscm and Tcm), essential for the long-term efficacy of CAR-T therapies, and mitigated CD8+ T cell loss, maintaining balanced CD4/CD8 ratios. These benefits make Optibumin an optimal choice for next-generation cell therapy manufacturing, offering a scalable, sustainable, and cost-effective solution that integrates seamlessly into existing workflows.
Materials and Methods
Cell Culture and Activation:
Primary human T cells were obtained from two healthy donors and prepared according to protocols designed to mimic workflows used in clinical administration of cell therapies. T cells were activated for 3 days using anti-CD3 and anti-CD28 T cell activators, followed by expansion for an additional 3 days from 2 × 10⁵ cells/mL to 1 × 10⁶ cells/mL by the time of cryopreservation.
Cryopreservation:
T cells were cryopreserved using CryoStor CS10 (10% DMSO) or CryoStor CS5 (5% DMSO) as the base cryopreservation solutions. The formulations were adjusted by adding 0%, 5%, or 10% human serum albumin (HSA) in the form of either Optibumin (recombinant HSA) or a clinically used blood-derived HSA. T cells were resuspended in the different formulations at a final concentration of 10 × 10⁶ cells/mL and incubated at room temperature for one hour before cryopreservation using a controlled rate freezing device (Mr. Frosty™). Controlled-rate freezing was used to ensure gradual cooling, promoting optimal ice crystal formation and minimizing cellular damage. After freezing, the cells were transferred to liquid nitrogen storage until thawing.
Thawing and Assessments:
To simulate typical cell therapy processing before administration (Li, 2019), cryovials were thawed, and the cells were incubated in cryopreservation formulations at room temperature for one hour before being plated in growth medium. Equal volumes of the thawed cells in their respective cryopreservation formulations were seeded into well plates for direct comparison of conditions. Viability and proliferation were determined using live-cell imaging techniques and specific staining protocols to differentiate between viable and dead cells.
Flow Cytometry:
At designated timepoints, plated cells were stained for viability using an amine-reactive dye and then subsequently stained with an optimized antibody cocktail. Analysis was performed by flow cytometry with a minimum live cell count of 10,000 per sample. Flow cytometry analysis began with gating for cell population based on forward scatter (FSC) versus side scatter (SSC) plots. Single cells were identified from FSC area versus FSC height plots. Dead cells were excluded based on high amine dye staining. Cells were then classified as either CD4+ or CD8+ populations using a 2D plot with a quadrant gate. Memory phenotypes were further defined based on CD45RA and CCR7 expression, as described by Tian et al (2017): Stem Cell Memory (Tscm) CCR7+ CD45RA+, Central Memory (Tcm) CCR7+ CD45RA-, Effector Memory (Tem) CCR7- CD45RA-, Terminal Effector Memory (Temra) CCR7- CD45RA+.
Footnotes
References
- Burnham, R. E., et al. (2021). Human serum albumin and chromatin condensation rescue ex vivo expanded γδ T cells from the effects of cryopreservation. Cryobiology, 99, 78–87. https://doi.org/10.1016/j.cryobiol.2021.01.011
- Van der Walle, C. F., et al. (2021). Formulation considerations for autologous T cell drug products. Pharmaceutics, 13(8), 1317. https://doi.org/10.3390/pharmaceutics13081317
- MacLennan, S., & Barbara, J. A. J. (2006). Risks and side effects of therapy with plasma and plasma fractions. Best Practice & Research Clinical Haematology, 19(1), 169–189. https://doi.org/10.1016/j.beha.2005.01.033
- Lu, H., Zhang, Y., & Liu, P. (2024). Identifying new safety risk of human serum albumin: A retrospective study of real-world data. Frontiers in Pharmacology, 15, 1319900. https://doi.org/10.3389/fphar.2024.1319900
- S. Food and Drug Administration. (2024). Considerations for the use of human-and animal-derived materials in the manufacture of cell and gene therapy and tissue-engineered medical products. U.S. Department of Health and Human Services, Center for Biologics Evaluation and Research. April 2024, Docket Number: FDA-2024-D-1244. https://www.regulations.gov/docket/FDA-2024-D-1244
- Madsen, B. K., et al. (2018). Adverse reactions of dimethyl sulfoxide in humans: A systematic review. F1000Research, 7. https://doi.org/10.12688/f1000research.16642.2
- Li, R., et al. (2019). Preservation of cell-based immunotherapies for clinical trials. Cytotherapy, 21(9), 943–957. https://doi.org/10.1016/j.jcyt.2019.07.004
- Fazeli, P., Kalani, M., & Hosseini, M. (2023). T memory stem cell characteristics in autoimmune diseases and their promising therapeutic values. Frontiers in Immunology, 14, 1204231. https://doi.org/10.3389/fimmu.2023.1204231
- Meyran, D., et al. (2023). TSTEM-like CAR-T cells exhibit improved persistence and tumor control compared with conventional CAR-T cells in preclinical models. Science Translational Medicine, 15(690), eabk1900. https://doi.org/10.1126/scitranslmed.abk1900
- Arcangeli, S., et al. (2022). CAR T cell manufacturing from naïve/stem memory T lymphocytes enhances antitumor responses while curtailing cytokine release syndrome. The Journal of Clinical Investigation, 132(12). https://doi.org/10.1172/JCI150807
- Lin, Y., Raje, N. S., Berdeja, J. G., Siegel, D. S., Jagannath, S., Madduri, D., et al. (2023). Idecabtagene vicleucel for relapsed and refractory multiple myeloma: Post hoc 18-month follow-up of a phase 1 trial. Nature Medicine, 29, 2286–2294. https://doi.org/10.1038/s41591-023-02496-0
- Das, R. K., et al. (2019). Naïve T-cell deficits at diagnosis and after chemotherapy impair cell therapy potential in pediatric cancers. Cancer Discovery, 9(4), 492–499. https://doi.org/10.1158/2159-8290.CD-18-1314
- Galli, E., et al. (2023). The CD4/CD8 ratio of infused CD19-CAR-T is a prognostic factor for efficacy and toxicity. British Journal of Haematology, 203(4), 564–570. https://doi.org/10.1111/bjh.19117
- Tian, Y., et al. (2017). Unique phenotypes and clonal expansions of human CD4 effector memory T cells re-expressing CD45RA. Nature Communications, 8(1), 1473. https://doi.org/10.1038/s41467-017-01728-5
Copyright © 2025 InVitria, Inc. All rights reserved.
Reproduction or distribution of any InVitria materials, in whole or in part, is prohibited without prior written consent. All logos, names, designs, and marks displayed, including InVitria®, the InVitria® brand design and Optibumin®, are trademarks or service marks owned or licensed by InVitria, Inc., Kansas, USA, unless otherwise noted. CryoStor® is a registered trademark of BioLife Solutions, Inc. Mr. Frosty® is a trademark owned by Nalge Nunc International Corp. For details on InVitria’s registered intellectual property, patents, and additional terms and conditions, please visit www.InVitria.com/terms.