- Home
- Lentivirus Production Protocol in Serum-Free Conditions – How To Improve Transfection Efficiency and Titer Yield
Lentivirus Production Protocol in Serum-Free Conditions – How To Improve Transfection Efficiency and Titer Yield
Published on 28 August 2021
Sofia Pezoa, PhD, Cell Culture Scientist, InVitria
Lentivirus Production in Serum-Free, Chemically Defined Conditions
Introduction
Viral vector-based gene therapies are promising candidates in the treatment of rare, genetic diseases due to the natural ability of viruses to transfer genetic content into target cells. Lentivirus (LV) is a propitious mechanism of genetic delivery due to the low immunogenicity of the virus, the ability to integrate into the genome, and the relative safety in the generation and handling of lentivirus. However, traditional production methods have relied on the use of fetal bovine serum in lentiviral production with adherent cells, raising the requirement for animal- and blood-free systems to overcome lot-to-lot inconsistencies, potential for adventitious agent contamination, and possible constraints in the serum supply chain.1 Therefore, we have developed a method for lentivirus production with adherent HEK-293T using OptiPEAK® HEK293T, a chemically defined, serum- and blood-free medium.
Background
Lentivirus falls into the class of retroviruses, which are enveloped, RNA-based viruses. While LV is derived from human immunodeficiency virus (HIV), LV is pseudotyped such that LV vectors lack the genes required to form an HIV particle. Furthermore, strategies to generate safer LV vectors and safer handling procedures have resulted in second and third generation vectors, separating the genes required to form the LV vector into either three or four plasmids that are transfected into the packaging cell. Lastly, LV vectors are replication-incompetent and generally self-inactivating, resulting in virus particles that cannot replicate themselves inside of a cell after delivering their genetic content. These advances in LV technology have resulted in safe and efficient vectors that can infect a wide variety of cells.
Clinical use of LV vectors for the treatment of rare genetic diseases or cancer is already underway with promising results.2 While Phase 3 clinical trials have not yet been completed, large scale manufacturing of LV vectors will be required to meet commercial demand of those products expected to be approved.
Traditional methods for LV production with adherent cells rely on the use of fetal bovine serum (FBS) for the growth and production phases of cells used to package LV vectors. FBS provides a source of growth factors and cytokines, as well as antioxidant capabilities; however, FBS in large scale manufacturing presents numerous constraints including but not limited to cost, lot-to-lot variation, and disruptions to the FBS supply chain. Furthermore, FBS also raises the possibility of the introduction of biological adventitious agents or contaminating substances that could impact the clinical efficacy of LV. Thus, there is a demand for a serum-free media that is commercially viable at scale. However, eliminating serum and blood-derived proteins for adherent HEK-293T cells have historically presented problems with the production, scalability, and stability of the produced viruses. Therefore, we have developed a serum-free, blood-free, and chemically defined media for the generation of high titer (>1 x 107 titer units per mL) LV vectors using adherent HEK-293T cells in commercial scale manufacturing.
Materials & Methods
Cell Lines and Reagents
HEK-293T (HEK-293T; ATCC CRL-3216) and HT-1080 (ATCC CCL-121) were maintained in either DMEM + 10% FBS for serum conditions, or complete OptiPEAK HEK293T (InVitria) for serum-free conditions (referred to as OptiPEAK HEK). Serum cultured cells were grown in standard conditions (5% CO2, 37 °C) while OptiPEAK HEK cultured cells were grown in 10% CO2 and 37 °C. Cells were adapted to serum free conditions by direct adaptation from FBS passaged cells. Both cell types were maintained by passaging with TrypLE (Thermo Fisher Scientific #A1217701) every 3-4 days and seeded at initial densities of 10,000 cells/cm2. HEK-293T cells were adapted to serum-free conditions over three passages. Optimum cell densities for seeding and transfection had been previously studied and documented.3
Transfection and Reagents
Second generation LV vectors were produced in adherent conditions in 2D using T-75 flasks with HEK-293T cells. For transfection, HEK-293T cells were seeded at a density of 75,000 cells/cm2 one day prior to transfection.
An optimized amount of plasmid DNA for transfection in both conditions was determined to be 0.16 µg/cm2 or 12 µg per T-75 flask. Second generation LV plasmids used were psPAX2 (Addgene #12260), pMD2.G (Addgene #12259), and pSIH1-H1-siLuc-copGFP transfer plasmid (System Biosciences). We selected an optimized ratio of 3 µg of transfer plasmid, 2 µg of VSV-g, and 1 µg of Gag-Pol, which has been reported to yield high titer LV vectors when compared to other ratios.4
For both serum-free and serum-containing conditions, the transfection reagent PEIpro (Polyplus) was used at a 1:1 ratio of plasmid DNA to PEI. For complexation, PEI and plasmid DNA were each first diluted 40-fold separately and gently mixed in either complete OptiPEAK HEK for serum-free or Opti-MEM™ (Gibco, Thermo Fisher Scientific) for serum conditions. Diluted plasmid DNA was then added to diluted PEI and mixed by gentle pipetting or swirling. Complexes were formed for 15 minutes at room temperature, then subsequently diluted further with the appropriate media to a final amount of 0.08 mL/cm2 and added to the cells by a complete media change after the conditioned media was aspirated from the flasks.
Cells were transfected for two hours at the described growth conditions, transfection complexes were removed by complete media change, and complete growth media was added back to the flasks at an amount equal to 0.13 mL/cm2. Transfected cells were then grown for 48 hours without media replacement, and the total supernatant was harvested from flasks and briefly centrifuged to clear cell debris, and then immediately used for functional testing.
At all steps, care should be taken to not dislodge cells from the tissue culture plastic.
Functional Titering
Functional titering of LV particles was performed as previously described.5 HT-1080 cells were plated in 12-well plates at a density of 30,000 cells per well and were allowed to adhere to tissue culture plastic for at least two hours. After attachment, the complete growth medium was replaced with transduction media consisting of DMEM + 10% FBS with 10 µg/mL Polybrene (Millipore Sigma TR-1003-G) and 0.1% F-68 (Thermo Fisher Scientific #24040032).
LV supernatant was serially diluted in infection media and then 5 µL of the diluted LV was added to cells. Cells were incubated for 48-72 hours and then harvested for fluorescence analysis by flow cytometry (BD Accuri C6 plus, BD). Functional titers were determined by using the following calculation:
Results and Conclusions
HEK-293T cells cultured in OptiPEAK HEK serum-free media show an average of 18.3% improvement in doubling times when compared to DMEM + 10% FBS (24.35 ± 2.54 hours in OptiPEAK HEK vs 29.81 ± 4.74 hours in complete DMEM). Morphological comparison of HEK-293T cells 27 hours post-transfection also showed equivalent characteristics in OptiPEAK HEK compared to serum (Figure 1).
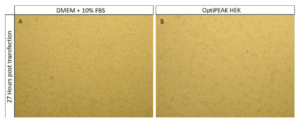
HEK-293T cells cultured in OptiPEAK HEK grow in a uniform monolayer and demonstrate the same morphological changes compared to DMEM + 10% FBS as HEK-293T cells begin to produce virus particles (Figure 1, B). These results suggest that transfection and viral production in serum- and blood-free OptiPEAK HEK proceed equivalently to serum conditions.
Analysis of functional titer by HT-1080 transduction assay yielded similar results to morphological characteristics and growth kinetics. Functional titers determined by flow cytometry in serum-containing and serum-free conditions showed equivalent performance (Figure 2). The average functional titer for DMEM + 10% FBS was 1.79 ± 1.03 x 107 TU/mL and the average titer for OptiPEAK HEK was 2.33 ± 1.91 x 107 TU/mL and falls within the standard deviation of DMEM + FBS (Figure 2). The data show equivalent performance for LV production in 2D with adherent HEK-293T cells in serum- and blood-free conditions compared to HEK-293T cells grown in the presence of 10% FBS.
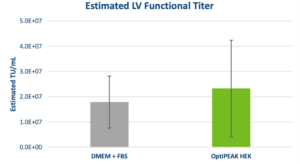
Clinical applications of LV require functional titers of >1 x 109 infectious units per patient which are only achieved after purification and concentration through either anion exchange chromatography or tangential flow filtration for large scale LV production.6 The above data show the results of applying a serum- and blood-free approach for LV production using adherent HEK-293T cells. This approach can be scaled up using adherent bioreactors such as the iCELLis® Bioreactor (Pall Corporation) and overcomes constraints from the FBS supply-chain and lot-to-lot inconsistencies.
Fixed bed bioreactors are paving the way for large-scale adherent based viral vector production, overcoming challenges faced in the scalability of adherent cell culture.6 Elimination of serum and blood-derived proteins in large-scale manufacturing will overcome numerous hurdles that often plague these manufacturing formats, such as foaming that is often observed with serum. Furthermore, while suspension culture has also been used to produce LV with HEK cells, it has only been successfully reported with production batch sizes less than 3 L.
We have demonstrated an approach for the production of LV with adherent HEK-293T cells in serum-free media that can be scaled up to production bioreactors with theoretical total yields of >1 x 109 TU/mL, meeting clinical demands without the constraints and inconsistencies associated with serum inclusion.
Footnotes
- Raturi, M and Andy Kusum.“The Blood Supply Management Amid the Covid-19 Outbreak”. Transfus Clin Biol 27 (2020).
- Milone, M. C. and U. O’Doherty (2018). “Clinical use of lentiviral vectors.” Leukemia 32(7): 1529-1541.
- Alfano, R., S. Pezoa, A. Pennybaker, and N. Hazi. “Application of Aber Biomass Probes to Inform Transfection Timing in Chemically Defined iCELLis® Bioreactor-Based Viral Vector Manufacturing,” (2021), Poster, invitria.com.
- Bajpai, R. (2011). “Lentivirus-Mediated Modification of Pluripotent Stem Cells,” in Human Pluripotent Stem Cells: Methods and Protocols, Methods in Molecular Biology, edited by P. H. Schwartz and R. L. Wesselschmidt, Springer Science + Business Media, LLC. 767: 315-331.
- Sena-Esteves, M. and G. Gao (2018). “Titration of Lentivirus Vectors.” Cold Spring Harb Protoc 2018(4).
- McCarron, A., M. Donnelley and D. Parsons (2017). “Scale-up of lentiviral vectors for gene therapy: advances and challenges.” Cell and Gene Therapy Insights 3(9): 719-729.